On February 7, 2019, Representative Alexandria Ocasio-Cortez introduced House Resolution 109 into consideration, “Recognizing the duty of the Federal Government to create a Green New Deal.” In order to combat climate change, the bill calls for “meeting 100 percent of the power demand in the United States through clean, renewable, and zero-emissions energy sources” (1). News coverage of the bill describes how it calls for a future powered enirely by renewable sources: solar, wind and hydroelectric (2). Although nonbinding and thus unable to spur any direct government action, the bill poses the question: in the face of climate change, what should the U.S. do to reduce greenhouse gas (GHG) emissions?
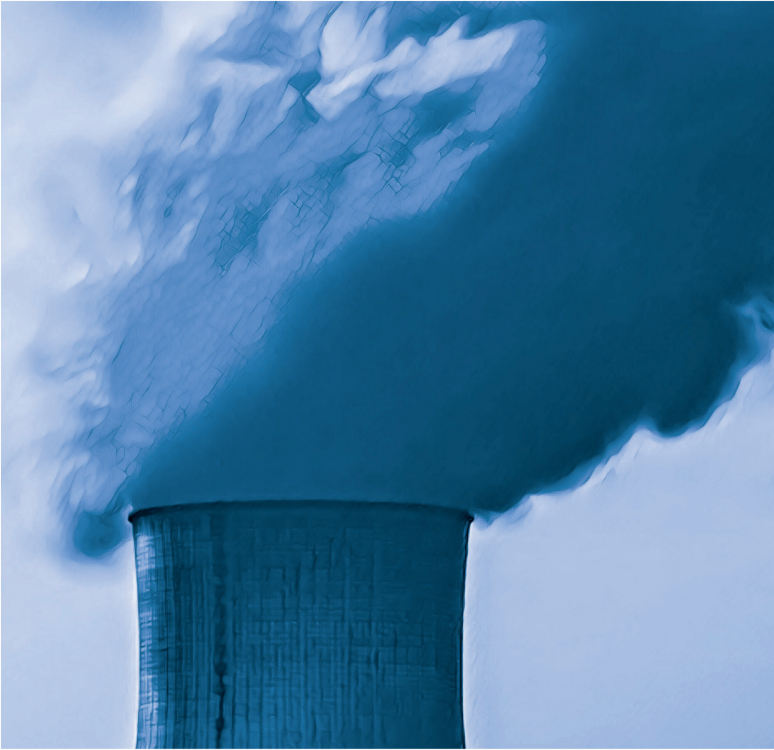
While not explicitly mentioned in the Green New Deal bill, there is a technology that has been proven effective at providing carbon-free energy reliably in multiple countries. This technology already supplies one-fifth of the U.S.’s electricity and over half of all its carbon-free electricity (3). It does so reliably and at low operational cost while subject to a strict regulatory system that imposes a high degree of safety. One might assume then, that the proponents of H.R. 109 would support the expansion of this technology, or at the very least its continued use at current levels. In fact, Rep. Ocasio-Cortez’s office states that their ultimate goal was “to transition away” from this technology (4).
The technology is nuclear power, and the contradiction between the goals of the Green New Deal and Rep. Ocasio-Cortez’s statement is indicative of the prevailing attitude towards nuclear power in the U.S. Despite the growing acceptance of the dangers posed by the continued use of fossil fuels, nuclear power does not feature prominently in proposals for new energy solutions (5). It is also considered risky and financially impractical. Advocates of renewable energies consider it unnecessary, for they believe that renewables alone can meet the demand for clean energy.
Contrary to these perceptions, nuclear power is necessary for eliminating U.S. carbon emissions as quickly as possible. Nuclear power can provide large amounts of carbon-free electricity more rapidly than renewable technologies, and it can do so with commercially viable technology, without geographic constraints, and without a huge change to the current U.S. electrical grid. It is true that the risks due to the radioactive content of nuclear waste are unique, but these risks are outweighed by nuclear power’s potential contributions to eliminate fossil fuel emissions. Despite negative public perception, nuclear power has an excellent record of operational safety. For these reasons, the U.S. government should commit itself to an expansion of nuclear power. The U.S. should build third-generation reactor designs already commercially viable in the U.S. and worldwide and provide incentives for licensing, financing, and construction of nuclear plants. Finally, to ensure the long-term viability of nuclear power generation, it is important for the U.S. government to encourage the development of novel nuclear technologies that produce less waste than current technologies. To meet this goal, the Nuclear Regulatory Commission (NRC) should provide a test site where prototypes of advanced reactor designs may be tested and licensed.
The motivation for a sweeping change in the sources of electricity in the U.S. is the need to drastically reduce carbon emissions in the next decade. The IPCC special report entitled Global Warming of 1.5oC concluded that limiting global warming to 1.5oC requires a 45% reduction of net carbon emissions from the 2010 baseline by 2030, and a total elimination of GHG emissions by 2050 (6). In their book A Bright Future: How Some Countries Have Solved Climate Change and the Rest Can Follow, Joshua Goldstein and Staffan Qvist describe the consequences of how passing climate “tipping points” can be even more extreme and unpredictable, including sea level rises of as much as 10-20 feet (7). Thus, reducing carbon emissions from electricity production as quickly as possible should be a central goal for the U.S.
The scale of the changes in energy production required to eliminate carbon emissions at the rates proposed by the IPCC are daunting. The historical emissions trajectory is nowhere close to meeting this goal. In fact, global carbon emissions grew by about 11% between 2010 and 2018 (7). In the Headline Statements of its report, the IPCC predicts that limiting warming requires “rapid and far-reaching transitions in energy, land, urban, and infrastructure…, and industrial systems” (5). To make the challenge more daunting, total U.S. electricity consumption per year is projected to grow by approximately 36% over the 2016 baseline by 2050 (8). Thus, carbon-neutral electricity sources must not only replace current fossil fuel sources but must also add extra capacity to meet this growth in demand. Based on measurements from the IAEA these emission-free technologies must generate over three times as much electricity as today to make U.S. electricity production carbon-neutral by 2050 (3).
Nuclear power can provide large amounts of carbon-free electricity more rapidly than renewable technologies, and it can do so with commercially viable technology, without geographic constraints, and without a huge change to the current U.S. electrical grid.
Nuclear power is a necessary technology for decarbonizing the U.S. electricity supply in accordance with the IPCC guidelines. With current technologies, nuclear power can produce carbon-neutral electricity anywhere in the U.S. Although their carbon footprint is comparable with renewable technologies such as solar, hydroelectric, and wind power plants, nuclear power plants are conceptually more similar to fossil fuel plants (9). In a nuclear plant, the amount of fuel alone determines the total electrical output. This is in contrast to renewable plants, which can be limited by geography, seasonal changes, or weather. Nuclear power plants can reliably reduce emissions by directly replacing fossil fuel plants, without the need for any further changes in the electrical grid.

In contrast, replacing fossil fuels with renewables—namely hydroelectric, solar, and wind power—is much less certain than doing so with nuclear power. The main limitation of renewable energy sources is the mismatch between their variable power outputs and demand for electricity. Making renewable technologies the primary source of electricity would require either the invention of unprecedentedly efficient long-term battery storage technologies or an overhaul of the electrical grid so that excess power from renewables can be used to make up for shortages from other sources, which would likely still fall short of U.S. energy needs (10-11). There have been many proposals for 100% renewable electricity grids, but these are even more limited by uncertainty in their ability to provide for future energy needs. Indeed, a review of 24 various plans for 100% electrical grids concluded that, “the case for feasibility [of 100%-renewable systems] is inadequate for the formation of responsible policy directed at responding to climate change” (12). This showcases that reducing emissions by expanding renewable technologies is more complex and less certain than replacing fossil fuel plants with nuclear plants.
Furthermore, historical evidence shows that nuclear power can add electrical generation capacity more quickly than any other carbon-neutral technology. A recent analysis of successful nuclear rollouts in Sweden and France during the 1960s-80s showed that Sweden added nuclear capacity at a sufficient rate that, if replicated worldwide, would totally replace global fossil fuels within 27 years (13). Moreover, this rate dwarfed recent attempts at large-scale national rollouts of renewable technologies elsewhere. For various countries, Goldstein and Qvist find that the carbon-free electricity added per person per year during the peak decade of nuclear power construction is on average larger than the peak decade of combined wind and solar construction. In a direct comparison, the electricity capacity added per GDP from the peak level of construction in Sweden is five times that of the peak wind and solar construction period in Germany (6). The reason for this discrepancy is that, although nuclear power plants can take several years to build, they produce more electricity per plant than renewable technologies and thus can quickly catch up with faster construction of less powerful renewable technologies. Moreover, the intermittency of renewable technologies requires that many more plants of equivalent capacity be built in order to achieve the same net power output as a single nuclear plant. The speed at which nuclear power adds emissions-free electricity makes it a critical technology for eliminating carbon emissions as quickly as possible.
This does not go to say that renewable technologies should not be expanded, nor that future technologies like battery storage might not eventually undermine the basis of the above arguments. Rather, it seems most responsible to consider the most probable path toward meeting the IPCC goals as soon as possible, and this path involves expanding not only renewables but also nuclear power. Put another way, allowing nuclear electrical generation in the U.S. to decline by 20% by 2050, which is currently predicted by the Energy Information Administration, means relying entirely on renewables and thereby greatly reduces the probability of meeting the IPCC goal (14).
What about the most common concerns regarding nuclear power: nuclear waste management and the operational safety of nuclear plants? These challenges are unique to nuclear power production, since no other energy technology uses highly radioactive fuel. Weighing that with nuclear power’s potential contribution to reducing carbon emissions, nuclear power should still be expanded in the U.S. The most difficult problem is the management of waste, but the risk associated with continued fossil fuel use and unmitigated climate change is greater than the risk associated with long-term geological storage of this waste.
Even though nuclear waste poses a serious and unique challenge, the potential harm to human society by not using nuclear power outweighs the dangers associated with waste.
High-level nuclear waste poses the most difficult challenge for nuclear power. Nuclear power plants transform their initial uranium oxide fuel into heavier elements, some of which are highly radioactive. The half-lives of some of these byproducts are incredibly long, meaning that a portion of the radioactive material will remain for an extended period of time. While most decay away within a few hundred years, a few have half-lives of up to 100,000 years (15). Although these radioactive byproducts constitute a very small percentage of the overall waste from a reactor, the fact that they remain dangerous for such an extremely long time poses a unique safety risk. Many countries, including the U.S., have adopted plans for disposal in deep geological repositories for long-term nuclear waste management, but no such repository for commercial waste is currently operational (16). Most commonly, due to safety concerns, local communities and political representatives resist placing waste disposal sites nearby. Moreover, the risk of the waste being removed from its containment site by chemical or geological activity cannot be predicted over the timescales during which the waste exists without large uncertainties (17). Early experience with waste sites in the U.S. is varied. In 2014, a drum of radioactive waste in the Waste Isolation Pilot Plant (WIPP) in New Mexico breached and released a small amount of high-level waste in the facility, leading to criticism of the site’s safety planning (18). The unsolved problem of geological waste disposal is arguably the most important reason for public opposition to nuclear power (17).

Even though nuclear waste poses a serious and unique challenge, the potential harm to human society by not using nuclear power outweighs the dangers associated with waste. Although all risk of future human contact with the nuclear waste cannot be entirely eliminated, geological repositories can be made very secure by redundant layers of containment. For instance, the Onkalo geological repository under construction in Olkiluoto in Finland, is based on a “multiple barriers” approach (19). The repository is embedded 400 meters deep in bedrock. The containment system involves putting the fuel in copper-iron canisters, putting each canister in an individual hole within the main deposition tunnel, filling both the holes and the main tunnels with non-permeable materials, and finally sealing off the entire repository (19). The main risk of nuclear waste is for a significant amount of high-level waste to permeate its containment and come in contact with humans in significant enough amounts to cause long-term health problems. While this possibility cannot be entirely ruled out for the thousands-year-long lifetimes of the nuclear waste facility, such an assembly should at least be judged to be secure for the foreseeable future.
Moreover, regarding the political challenge of choosing a repository site, Finland’s repository selection process provides a model for the U.S. In selecting sites, the company in charge of the site selection consulted the local communities near the sites and gave each community veto power over their site being selected (20). This approach increased public understanding and trust in the repository. Adopting these strategies could improve the political viability of long-term geological disposal in the U.S.
It is also worth mentioning that developing technologies could reduce the waste burden of nuclear power. In particular, thorium reactors, which are currently being explored in India and China, generate significantly less radioactive waste than uranium reactors, which is the current style of most power reactors (21). Indeed, the waste from thorium reactors must be stored for only about 500 years, compared to tens of thousands of years for waste from uranium reactors (22). However, thorium reactors are not yet commercially viable, and thus an expansion of nuclear power in the U.S. necessary to meet the IPCC goals must rely in large part on current reactor designs. However, just as the intermittency of renewable technologies might be addressed by new battery technologies, next-generation nuclear technologies may significantly reduce the problem of nuclear waste management.
Finally, although a rapid expansion of nuclear power would most easily be accomplished with already licensed reactor designs, the U.S. government should promote the prototyping in licensing of advanced reactor designs.
Finally, contrary to public perception, the operation of nuclear power plants is quite safe both for workers at the plant and the general public. The main safety concern for operational nuclear plants is that, if a plant loses power, the heat produced by the radioactive fuel could destroy the reactor core and release radioactive material into the air. Given the dangers of radioactive release, it is reasonable to demand high safety standards for nuclear reactors. However, all available evidence shows that these standards are met, both in the U.S. and globally. According to data compiled by the earth scientist James Conca in 2012, the number of deaths per trillion-kW hour of electricity produced globally by nuclear power was 90, including estimates for Chernobyl and Fukushima, compared with 150 for wind power and nearly 4,000 from natural gas (23). While these numbers rely on epidemiological assumptions, the main point is that the nuclear reactors currently in operation are relatively very safe. Even in the case of serious accidents, modern reactors are safer than is currently believed. According to the World Health Organization, no significant health effects from radiation released at the Fukushima nuclear plant have been documented, even among those with the most radiation exposure (24). Finally, advanced reactor designs currently under construction, such as the Westinghouse AP1000 reactor, involve passive safety features—safety mechanisms that do not have to be actively triggered (25). A recent study by the Massachusetts Institute of Technology (MIT) Energy Initiative entitled The Future of Nuclear Energy in a Carbon-Constrained World found that these mechanisms are already commercially viable in plants in operation in China, Russia, and the U.S. (26).
Nuclear power is critical to reducing carbon emissions to the level stated in the IPCC recommendations. This fact justifies an expanded use of nuclear power, despite the remaining challenges of nuclear waste management and safety. What, then, should the U.S. do to expand nuclear power in order to meaningfully reduce carbon emissions?
First, the U.S. government should invest directly in the construction of nuclear plants and provide incentives for zero-emissions electricity production. In the private sector, the large upfront costs of nuclear power and the long construction times for nuclear plants make nuclear less attractive for investment than cheaper fossil fuels, especially natural gas. By funding the construction of plants, the government would remove this obstacle from nuclear power expansion. Moreover, the idea that nuclear power will be consistently expensive is based largely on analysis of the “negative learning curve” experienced in the U.S. and France during the 1970s (27). However, a more recent economic analysis shows that, in South Korea, repeated builds of a standardized design became successively less expensive (28). For example, the U.S. could adopt Generation III designs such as the Westinghouse AP1000 or the South Korean APR 1400 and commit to building them repeatedly. This approach would avoid the expense and delays that have plagued AP1000 in the U.S. (29). This approach is also recommended by Goldstein and Qvist (6).
Finally, advanced reactor designs currently under construction, such as the Westinghouse AP1000 reactor, involve passive safety features—safety mechanisms that do not have to be actively triggered.
Aside from direct investment, the government could make nuclear power more financially attractive by compensating nuclear plants for their lack of carbon emissions. As indicated by Daniel Shea and Kristy Hartman, writing for the National Conference of State Legislatures, New York and Illinois offer “Zero Emissions Credits” that help keep older nuclear plants open (30). Federal and state versions of these credits would make investing in nuclear power more profitable. Shea and Hartman also point out that most states that incentivize renewable energy do not offer similar incentives to nuclear power. These states should rectify this inconsistency in order to incentivize private investment in nuclear power. Moreover, to further this goal, the NRC should adopt a licensing framework that enables greater standardization of reactor design. As discussed in the recent MIT study on the future of nuclear technology, this standardization would reduce the uncertainty of nuclear projects and make them more attractive to future investments (26).
Finally, although a rapid expansion of nuclear power would most easily be accomplished with already licensed reactor designs, the U.S. government should promote the prototyping in licensing of advanced reactor designs. As recommended by the recent MIT study, the government should “establish reactor parks where companies can site prototype reactors,” as well as “fund prototype testing and commercial deployment of new, advanced reactor designs” (26). These actions will speed up the commercial viability of advanced reactors without sacrificing the strictness of the licensing procedure. Having advanced reactors available before 2050 will also prevent technological lock-in during a nuclear power expansion. However, the bulk of any expansion of nuclear power should depend on nuclear plant designs that have been constructed or are under construction.
It is imperative for the U.S. to reduce carbon emissions. This action will not only contribute to limiting climate change, but will also make the U.S. a leader on a crucial worldwide priority. Nuclear power is able to provide a large percentage of the U.S. electricity with current technology within the current electrical grid. Historical precedents show that this technology can be scaled up before 2050, and nuclear power is safer than is often believed. Consequently, the U.S. should both fund public construction and incentivize private construction of nuclear power plants.
- House Resolution 109, 116th Cong. (2019).
- Geuss, M. (2019, February 07). Green New Deal bill aims to move US to 100% renewable energy, net-zero emissions.
- U.S. Energy Information Administration - EIA - Independent Statistics and Analysis. (n.d.). Retrieved from https://www.eia.gov/tools/faqs/faq. php?id=427&t=3
- Kurtzleben, D. (2019, February 07). Rep. Alexandria Ocasio-Cortez Releases Green New Deal Outline.
- IPCC (2018). Global Warming of 1.5°C. An IPCC Special Report on the impacts of global warming of 1.5°C above pre-industrial levels and related global greenhouse gas emission pathways, in the context of strengthening the global response to the threat of climate change, sustainable development, and efforts to eradicate poverty.
- Goldstein, J. S., & Qvist, S. A. (2019). A bright future: How some countries have solved climate change and the rest can follow. New York: PublicAffairs.
- Quéré, C. L. et al. (2018). Global Carbon Budget 2018. Earth System Science Data,10(4), 2141-2194.
- Steinberg, D. et al. (2017). Electrification & Decarbonization: Exploring U.S. Energy Use and Greenhouse Gas Emissions in Scenarios with Widespread Electrification and Power Sector Decarbonization (NREL/TP-6A20-68214). National Renewable Energy Laboratory.
- Pehl, M., Arvesen, A., Humpenöder, F., Popp, A., Hertwich, E. G., & Luderer, G. (2017). Understanding future emissions from low-carbon power systems by integration of life-cycle assessment and integrated energy modelling. Nature Energy,2(12), 939-945.
- Clack, C. T. et al. (2017). Evaluation of a proposal for reliable low-cost grid power with 100% wind, water, and solar. Proceedings of the National Academy of Sciences,114(26), 6722-6727.
- Macdonald, A. E., Clack, C. T., Alexander, A., Dunbar, A., Wilczak, J., & Xie, Y. (2016). Future cost- competitive electricity systems and their impact on US CO2 emissions. Nature Climate Change,6(5), 526-531.
- Heard, B., Brook, B., Wigley, T., & Bradshaw, C. (2017). Burden of proof: A comprehensive review of the feasibility of 100% renewable-electricity systems. Renewable and Sustainable Energy Reviews, 76, 1122-1133.
- Qvist, S. A., & Brook, B. W. (2015). Potential for Worldwide Displacement of Fossil-Fuel Electricity by Nuclear Energy in Three Decades Based on Extrapolation of Regional Deployment Data. Plos One,10(5).
- U.S. Energy Information Administration (2018). Nuclear Power Outlook 2018.
- Hedin, A (1997). Spent nuclear fuel—how dangerous is it? A report from the project “Description of risk” (Tech. Rep. 97-13). Swedish Nuclear Fuel and Waste Management Co, Stockholm, Sweden.
- Report of the Secretary of Energy (2012). Blue Ribbon Commission on America’s Energy Future.
- Ramana, M. V. (2018). Technical and social problems of nuclear waste. Wiley Interdisciplinary Reviews: Energy and Environment,7(4).
- Tracy, C. L., Dustin, M. K., & Ewing, R. C. (2016). Policy: Reassess New Mexico’s nuclear-easte repository. Nature,529(7585), 149-151.
- Posiva Oy (2012). Safety Case for the Disposal of Spent Nuclear Fuel at Olkiluoto. (Rep. 2012-08).
- Fountain, H. (2017, June 9). On Nuclear Waste, Finland Shows U.S. How It Can Be Done. The New York Times.
- Katusa, M. (2012, July 17). The Thing About Thorium: Why The Better Nuclear Fuel May Not Get A Chance.
- Schaffer, M. B. (2013) Abundant thorium as an alternative nuclear fuel: Important waste disposal and weapon proliferation advantages. Energy Policy, 60 4-12.
- Conca, J. (2017, March 28). How Deadly Is Your Kilowatt? We Rank The Killer Energy Sources.
- United Nations Scientific Committee on the Effects of Atomic Radiation (UNSCEAR) (2015). Developments since the 2013 UNSCEAR Report on the Levels and Effects of Radiation Exposure Due to the Nuclear Accident Following the Great East-Japan Earthquake and Tsunami: A 2015 White Paper to guide the Scientific Committee’s Future Program of Work. United Nations, New York.
- New Plants. (n.d.). Retrieved from http://www. westinghousenuclear.com/New-Plants/AP1000-PWR
- Buongiorno, J. et al., M.I.T. Energy Initiative (2018). The Future of Nuclear Energy in a Carbon-Constrained World: An Interdisciplinary MIT Study. M.I.T.
- Grubler, A. (2010). The costs of the French nuclear scale-up: A case of negative learning by doing. Energy Policy,38(9), 5174-5188.
- Lovering, J. R., Yip, A., & Nordhaus, T. (2016). Historical construction costs of global nuclear power reactors. Energy Policy, 91, 371-382.
- Plumer, B. (2017, July 31). U.S. Nuclear Comeback Stalls as Two Reactors are Abandoned. The New York Times. Retrieved from
- Shea, D. & Hartman, K. (2017). State options to keep nuclear in the energy mix. National Conference of State Legislatures.